Collagen Proteins - A Fibrous Family That Supports Life
In biochemistry, perhaps the most abundant and varied family of molecules we know of are the proteins. This is certainly true for cellular biology, with the typical cell being able to hold some 42 million proteins in its cytoplasm at each point in time. Proteins are not just muscle-builders - they are the most principal machinery the world of living cells use to function. They include enzymes, DNA expression factors (known as transcription factors), transporters, locomotive cytoskeleton filaments, and a whole bunch of other important members that we observe all over the cell.
Incredibly, cells are nonetheless able to precisely coordinate the many pathways involving the millions of proteins working within them. Though it is difficult to accurately portray such a situation, we might try to imagine it like this. How would it be to manage an entire nation with a population of 42 million people, optimally handling all the national crime, education, welfare and hygiene for all the areas covering it 24/7? Cells would know - each of the trillions that make up our bodies.
Outside the cells, we also find spaces filled with proteins in multicellular organisms. Such spaces might bind cells together, allow absorbed nutrients to diffuse into the cells more easily, or even just provide structural support to the organism as a whole. We call this space the extracellular matrix (ECM) - and it includes the bone matrix, blood plasma, tissue fluid and our tendons. Although these also contain a multitude of mind-bogglingly complex protein families, there is one family that we find almost universally present in our bodies. It is known as the collagen family. You may have heard of these marketed in hair products and other sorts of cosmetic products, and perhaps even that they can promote the rejuvenation of youth. Unfortunately, much of this is exaggerated, and does not really hold true for the science behind collagen. Albeit, there are some fascinating properties associated with collagen proteins, as we shall find out soon. First, however, let's take a look at what these molecules are even made of.
A Rope-Like Structure & Stability
So far, researchers have found 28 different types of collagen in biological organisms. These are designated with Roman numerals - ranging from collagen I to collagen XXVIII - but their amounts vary between species. In humans, for instance, we find that 90% of our collagen is found exclusively as collagen I and collagen IV. The difference between these is subtle; while both have important roles in our internal structural support, one might consist of a few different amino acids that allows it to form more easily in a dissimilar medium in the body (e.g., the bone matrix contains mostly collagen I, with most of the basement membranes that surround or tissues comprised of collagen IV). Collagen strands are also known to generally be over 1,400 amino acids long, making them rather gigantic molecules. It is admittedly a bit vague to say that these structures differ by 'amino acid', however, so let's go into that in a bit more detail.
Like all polypeptides (i.e., proteins), collagens are first formed in specialised cells called fibroblasts from a sequence of 20 standard amino acids, which are later modified to construct their three-dimensional shape in the ECM. Since collagens are fibrous/linear proteins, this means that their amino acid sequences are regularly organised to produce the repeated triple helix coils that make them. You might imagine one as a rope, with three interacting fibres wrapping around each other to provide the protein with tensile strength. Analysing the specific structure of human collagen, scientists have further found certain amino acids recurring in essentially equal intervals between them - such as glycine, proline and leucine - all of which provide specialised properties to the protein. This becomes more apparent after imaging fully matured collagen I using modern imaging techniques like X-ray crystallography. In this way, researchers have found glycine residues to occur every third amino acid in the centre of its rope-like structure, with proline residues appearing on its outside. Why is this?
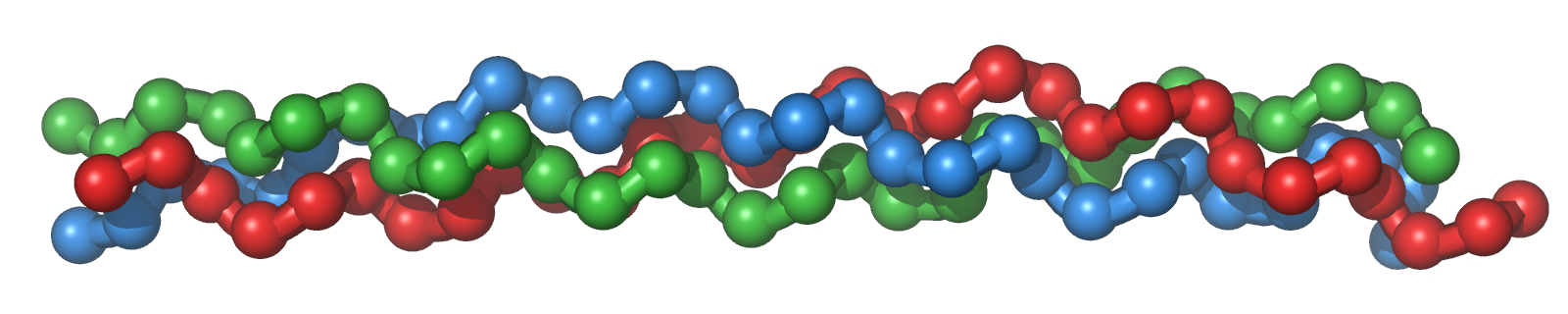
All amino acids have a general structure assigned to them; they include a carbon atom bonded to an amine group (NH2), a carboxyl group (COOH), a hydrogen atom and a side chain unique to the corresponding amino acid. In polypeptides, all of these elements are significant in producing their resulting 3D structure in space. The amine and carboxyl groups, for example, are useful for establishing intermolecular hydrogen bonds (defined as weak electrostatic attrations between partially charged atoms, such as between hydrogen and oxygen atoms) between different chains. This is what allows certain polypeptides to form coils, as their specific amino acids are oriented in just the right way to attract each other into such a structure.
In turn, it is the amino acids' side chains that result in the specific orientations allowed in collagen chains. Glycine, for one, is the simplest amino acid there is, containing only another hydrogen atom in place of a side chain. This also means that it is very small - enough that, in a protein structure, it can be used to fill in the gaps too narrow for other amino acids to be in. Since collagen exists in an aqueous environment, which interacts favourably with polar solvents (and not so much with non-polar molecules), we must also consider the polarity of its constituents. While all amino acids have polarity in their backbone, it can vary in their side chains. Again, such is the case with glycine; given its simple structure, it does not display as much polarity nor attraction to polar solvents (i.e., water) as other amino acids. In turn, glycine residues can be hidden away from the surrounding ECM in the middle of a fibrous protein, and so we obtain the tightly packed triple helix that we see in collagen.
Other non-polar amino acids lie somewhere in the middle, with polar residues found mainly facing the aqueous environment (the ECM, in the case of mature collagen). Similarly, proline can usually be found modified into hydroxyproline to make it more soluble in water and more stable at the outside of a collagen fibre. This particular modification occurs in humans using hydroxylase enzymes, during the time in which the protein is premature and has only just been synthesised in the cytoplasm of a fibroblast cell. Interestingly, the hydroxylases involved require vitamin C as a cofactor to work, resulting in the disruption of collagen production and all sorts of structural problems without it. For exmaple, scurvy - a condition characterised by weak blood vessel walls, frail skin and slow injury healing - is a good example of how body collagen is affected by vitamin C deficiencies. So, in other words, we don't only need dietary proteins to build muscle and a healthy physique - we need fruits and vegetables, too!
Another benefit of collagen is how chemically stable it is in the tumultuous environments of biological organisms. In humans, researchers have even found that collagen I fibres could continue existing without being degraded and broken down for around 20 years, after which they could simply be replaced by new fibres. This is also considering that, in our bodies, there are many systems in place designed to degrade and recycle our proteins into more suitable versions over time. Outside the body, this then becomes an entirely different story, with collagen being found intact in even the remains of dinosaurs from 195 million years ago. Without the chaotic reactions and protein-degrading enzymes in life, collagen is simply too unreactive to break down by itself (though it is still very biodegradable, of course, as bacteria and other decomposers are more than happy to have a bit of collagen!). Nonetheless, high temperatures can massively accelerate this process, as we see when cooking meals from protein- and sugar-containing ingredients.
Collagen is a useful material in life. It builds and supports us, and it gives shape to the dynamic processes that allow us to survive. There are other ways to take advantage of its properties, however - as both amphibians and humans have realised.
Using Collagen For Growth, Medicine & Health
Given that collagen is a polymer made of individual monomer units, it also has the incredibly useful property of being flexible to change. This is particularly obvious in most amphibians, as we see in the remarkable whole-body change that frogs experience during their metamorphosis from young tadpoles to adult frogs. In contrast, a select few amphibians have subsequently ditched this function via natural selection some millions of years ago, with axolotls in particular now simply remaining aquatic salamanders throughout their lives. There is much to learn about these processes, and collagen metabolism is key to their mechanisms.
There are other things we can do with collagen. Biomedical applications of the proteins have been under talks for the past two decades or so, as scientists have argued for collagen's uses in things like:
- Drug delivery systems due to their biocompatibility with an organism
- Tissue engineering, including skin, bone and cardiovascular tissue replacement
- Manufacturing analogue ECM environments as stem cell growing platforms for artificial organ synthesis
In regards to its uses in cosmetic and skin health products, well...scientists are not sure. Since the appearance of aging skin is really due to a shortage of collagen in the dermis as you age, it does seem like a good idea to apply cream that contains collagen precursors that can be absorbed by the skin. However, there have not been sufficient experiments to prove this. There have been a few studies that showed a link between oral collagen consumption and increased skin hydration and elasticity, and there does not seem to be adverse effects to applying their cream variants. So, if you feel better doing it, by all means - use your moisturisers. If you really want to have healthier skin and improve your collagen storages, however, I would advise using sunscreen. High-energy radiation from the sun can damage collagen fibres, and we want to stop that from happening first and foremost!
As always, there is much research to be done on the matter. Collagens are fascinating proteins, and they probably offer the most support in animal bodies out of anything else. It makes sense that nature uses them almost everywhere, then!
References
- University of Toronto (2018). A cell holds 42 million protein molecules, scientists reveal. ScienceDaily. Retrieved from https://www.sciencedaily.com/releases/2018/01/180117131202.htm
- Wu, M. & Cronin, K. & Crane, J. S. (2021). Biochemistry, Collagen Synthesis. StatPearls. Retrieved from https://www.ncbi.nlm.nih.gov/books/NBK507709/
- Lee, C. H. & Singla, A. & Lee, Y. (2001). Biomedical applications of collagen. International Journal of Pharmaceutics 221(1-2). Retrieved from https://doi.org/10.1016/S0378-5173(01)00691-3
- Sorushanova, A. et al (2018). The Collagen Suprafamily: From Biosynthesis to Advanced Biomaterial Development. Advanced Materials 31(1). Retrieved from https://doi.org/10.1002/adma.201801651
- Goh, K. L. & Holmes, D. F. (2017). Collagenous Extracellular Matrix Biomaterials for Tissue Engineering: Lessons from the Common Sea Urchin Tissue. International Journal of Molecular Sciences 25;18(5):901. Retrieved from https://doi.org/10.3390/ijms18050901