Turbulent Waters - A Driving Force For The Origin Of Life
Even before life, there was chemistry. Molecules like carbon dioxide, methane, water, etc. floated around Earth's atmosphere and swirled in the oceans below, occasionally bumping into each other to bond, change, or otherwise break apart. Somehow, these seemingly trivial dynamics led to the origin of life. Whether they were proteins, DNA or RNA - each a crucial component to life as it is now - our all-time ancestor for self-replication and metabolic reactions would have been made from common building blocks. As RNA currently seems the most likely candidate for kickstarting biology (though it is not set in stone), such building blocks were likely nucleotides, the basic units for nucleic acids. All biomolecules feature a backbone of carbon atoms, however, so we can be sure that life was the result of stochastic organic chemistry pathways in their environment.
Over time, chemists and biochemists have worked together to solve the mystery behind the prebiotic chemistry responsible for life. Already, we have discovered a remarkable variety of synthetic routes that could have been involved in the phenomenon. A recent study, for example, demonstrated how simple organic molecules could combine together and with phosphate ions to obtain all the nucleotides needed to build RNA, while another gave evidence for a plausible analogue to the Krebs cycle made exclusively of α-ketoacids. The Krebs cycle, I should note, is the main reaction network that living cells use to recycle metabolic compounds for the production of biomolecules or energy. It occurs in the cells' mitochondria - small compartments of metabolites that keep the energy-generating reactions separated from the cytoplasm - and it requires the help of dozens of interacting enzymes to work. We may be surprised to hear, then, that the prebiotic world could have done anything similar on its own.
This is the crux and dilemma that we find ourselves in; how on earth could a prebiotic, lifeless and rocky environment become sufficiently organised to produce such a sophisticated network by itself? The question becomes especially confounding when you realise that the universe isn't quite keen on order, to say nothing of the chaotic nature of chemical reactions. In an interview with Dr Kamila Muchowska, CNRS fellow and prebiotic chemistry researcher from the University of Strasbourg, she described it as an ongoing challenge in the scientific community.
"The problem of 'origin of life' studies is finding a way in which these [disorganised] materials self-organised into something that looks very structured, even while still in a dynamic system," Muchowska explained. Fortunately, though, we are now starting to see some light at the end the tunnel.
A Lifeless Metabolism
To study the details behind prebiotic chemistry, it easier to deal with an imagined replica of the scenario than with the event itself. It is a slight compromise that scientists must make, given that we can't go back in time quite just yet. But people have become rather adept at mimicking natural events - or, in this case, at least at drawing up a near-enough rendition of them - so we are still able to extract much valuable information from such models.
For our purpose, the model systems we're after typically have some route that leads to the aforementioned biomolecules. One possible precursor to nucleic acids, in this case, is formaldehyde. Made of one carbon atom covalently bonded to two hydrogen atoms and one oxygen, formaldehyde is one of the simplest organic molecules we know of, right behind methane and methanol. It is also highly reactive, as a result of the unequal dispersion of electrons between the carbon and oxygen atoms inside it. In biology, the more reactive the biomolecule, the easier it can pass chemicals from one compound to another within a cell...sometimes detrimentally. Formaldehyde has consequently been shown to have exceptional abilities as both a single-carbon carrier and a colourless yet pungent poisonous gas. Understandably, researchers are not fond of letting it spread too much - and not just because of the waste in material!
When dissolved in solution, formaldehyde has been observed to react not only with other chemicals, but also with itself. The molecules can then react together again to produce even longer or more convoluted carbon chains, some of which proceed to break apart from instability and restart the system from a different direction. Widely known as the formose reaction (and less widely as the Butlerov reaction), this chemical network was first discovered in 1861 by Russian chemist Aleksandr Butlerov. Prior to this, Butlerov had also discovered formaldehyde and proposed the existence of the tetrahedral geometry in carbon compounds; achievements that ultimately got his name attached to a moon crater by NASA in 1982. (How would he have felt about that, I wonder?)
When testing out the behaviour of dissolved formaldehyde, Butlerov found that it stimulated what he called a 'sugary substance' to emerge. Through extensive analyses of the network, we now know that this was simply due to the tetrose sugars made in the later steps of the formose reaction, the cyclic mechanism of which was partly unearthed in 1959 by American chemist Ronald Breslow and by others thereafter.
Scientists have similarly identified an assortment of other molecules in the system, letting them tweak the formose reaction at the molecular level and compare it with metabolic pathways in cells. Some compounds worth noting here are glyceraldehyde and dihydroxyacetone, both involved in the first part of cellular respiration; and ribose, a five-carbon sugar that forms the core of RNA. As Muchowska puts it: "The formose reaction is extremely important from a mechanistic perspective, not just because we want to make sugars, but [because it tells us] how networks work in general."
All in all, it would seem like there shouldn't be much left to discover from the formose reaction, especially with other model systems like the reverse Krebs cycle providing much material to explore. Nonetheless, a team of researchers from Radboud University, in the Netherlands, recently uncovered a major aspect of the system - and indeed of all prebiotic chemistry - previously left entirely unnoticed.
Organised Turmoil
What happens when you leave dilute raw honey to sit? If you've ever stored some for long periods before, you might know that it tends to thicken and solidify with time, forming separate liquid and crystal sections in a jar. After that, the honey might also spoil; but we'll pretend that microbes don't exist for the time being. In this hyper-sterile environment we're imagining, raw honey behaves, rather interestingly, a bit like a solution encompassing the formose reaction. When left alone in constant conditions, such a solution is also liable to thicken, this time more quickly and into a viscous tar-like substance. Whereas honey can be heated and stirred back into its delicious self, however, tar produced from the reaction is practically irrevocable (and a tad less edible). More to the point, it is quite unexciting for scientific research.
Besides adding minerals and compounds to mimic underwater environments in the past (e.g., calcium ions usually represent the dissolution of calcium deposits on the sea floor), previous studies on the subject have all let the formose reaction occur without allowing any significant fluctuations in its parameters. This is standard practice in the sciences, letting researchers compare the effects of different experiments on identical solutions. And it works - for the most part. Regrettably, keeping conditions constant leads to tar, and it doesn't provide information about how the chemistry 4 billion years ago could have been sufficiently organised to drive the complex formation of life. So how do we address this?
This year, a group led by Professor Wilhelm Huck at Radboud University centered a study in Nature on exactly this question. To tackle it, they used a special flow reactor (essentially a fancy container where liquids can flow into and out of it at specified rates) to continually mix a solution with water and reactants and minerals while simultaneously pumping the products out. Besides the water inlet, the reactor had a conduit for calcium ions and sodium hydroxide; one for formaldehyde; and another to add one of four starting sugars for the reaction. Throughout the operation, they then altered a total of 17 environmental variables to further emulate turbulent conditions. Some of these included the temperature of the solution, the concentration of the chemicals used, which starting sugar was added, and the speed at which the liquids flowed through the reactor. Needless to say, this resulted in a gigantic number of combinations.
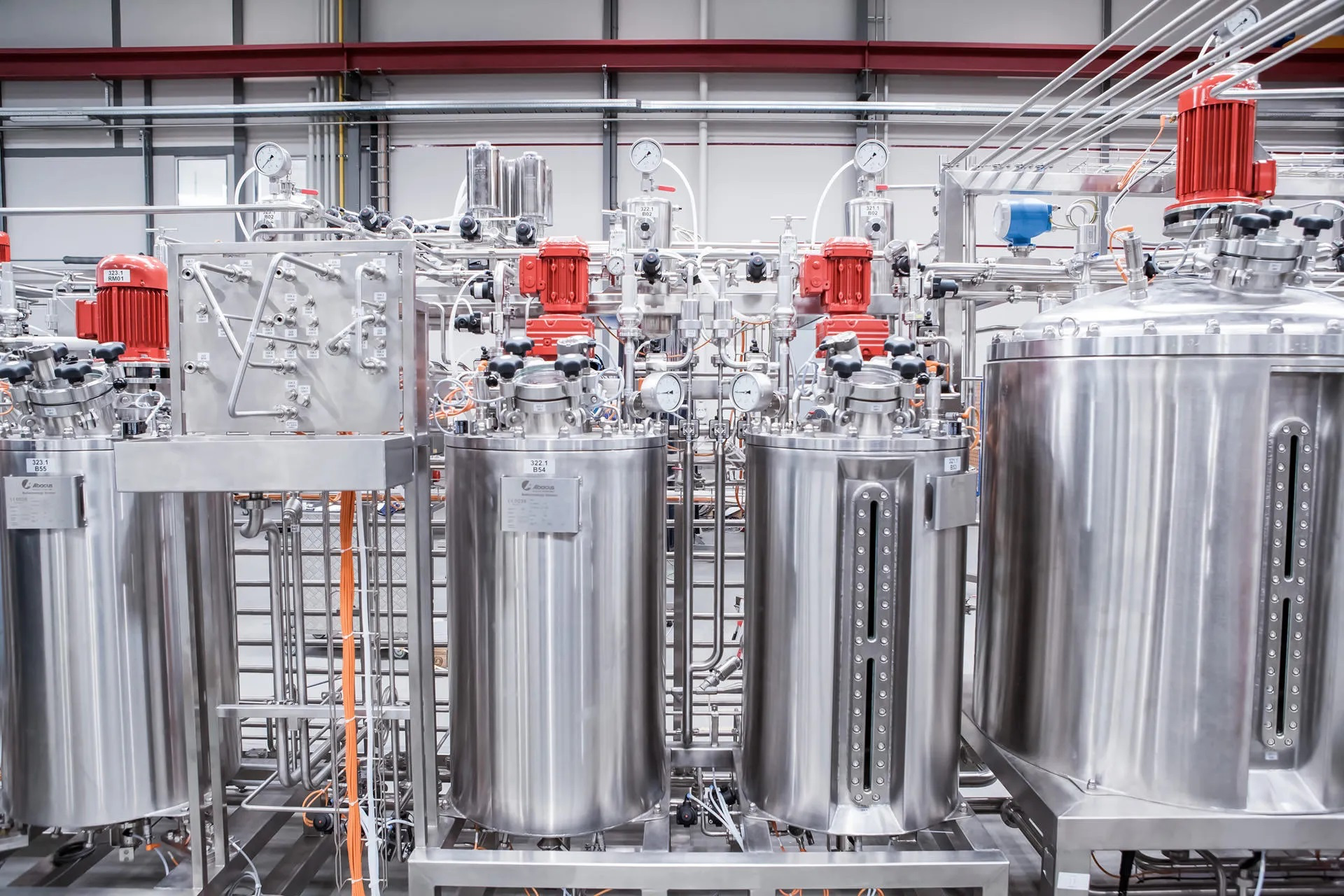
"Up until now the predominant objective [in prebiotic chemistry] has been to try to synthesise the building blocks in the origin of life, given what was available," says Dr William Robinson, lead-author of the paper and postdoc in the Huck Group. "But life isn't just a series of chemicals coming together...What we were really thinking was, how can we make this chemistry more dynamic?" Importantly, the outflow of the flow reactor was continuously sampled and tested for its composition, seen to change according to the parameters used at each time.
Given the massive amount of possible variation in the system, it would have been practically impossible to derive any meaningful data from the results by comparing them manually. Thankfully, though, modern technology has been developing rapidly in the field of research. Consequently, the team was able to use data mining techniques to quickly sort out all the products found in their composition sampling and match them up against their respective reaction conditions, displaying the results in a scientific tree diagram (more formally referred to as a dendrogram). Here was the kicker of the story.
"The interesting thing that we found was that the [formose reaction] actually generates its own reactants - it makes its own reaction pathways - which has a surprising effect on what we observe," mentioned Robinson. Further scrutiny saw a pattern in the whole.
From the seemingly haphazard and indecipherable mess that was the formose reaction, the researchers found that several pathways in the network occurred more frequently than others. More specifically, the types of chemical reactions present appeared to shift according to the environmental conditions, with many similarly conditioned pathways leading to analogous product compositions. When the formaldehyde concentration in the flow reactor was lower, for example, the network tended to favour addition reactions between 2- and 3-carbon molecules to obtain larger organic products. Furthermore, increasing the ratio of calcium to sodium ions in the mixture promoted the creation of some 4- and 5-carbon sugars, including erythrose and ribulose - both of which are important in nucleotide synthesis in cells. Calcium ions also appeared to stabilise particular orientations of some compounds, hinting at a possible mechanism for how the nigh-omnipresent left-handedness of biological molecules first came to be.
Perhaps unsurprisingly, the tree diagram's branches diverged significantly when the starting sugars used were different. "Depending on where you start, you end up in a different place," remarked Robinson. "In some cases there's crossover in the kinds of compounds that are made, and this allowed us to explore that space." Comparatively, changing the temperature did not affect product compositions very much at all. The rest of the factors influenced the results in their own accord; and we could go through these one by one. Albeit, we have already discussed some of the more conspicuous changes to the system, and in any case the overall picture remains the same. There are patterns in the disorder of the formose reaction, many of which become more apparent in fluctuating surroundings. The system, in other words, self-organises.
"It's almost like a code in the environment and the compounds in it, which can create specific compositions," explained Robinson. It should be noted that, as alluring as it is to believe that the formose reaction was the key to the origin of life, there are other model systems that could have been the same. As such, the significance of their paper focuses more on the implications it creates for future research.
"I don't believe that this study is the definite answer...for how sugars first appeared," Muchowska elaborated, "but it's extremely important in that it teaches us how chemical networks function." Clearly, while anaylising a single model might not reveal all the secrets to prebiotic chemistry, the ability to build upon multiple models simultaneously is often just as valuable. That said, there is still one side of the study that we haven't explored, the likes of which may just point to the formose reaction as a likely precursor for cell metabolism. For that, we must return to Ronald Breslow.
The First Biochemical Cycle
When Breslow first proposed a mechanism for the formose reaction, it was with a single idea in mind: the network was probably autocatalytic. I mentioned it before, but this would have meant that some constituent reaction in the network causes the same reaction to occur again, in turn forming a cycle of the ordeal. Kind of like how a blacksmith might use a hammer and steel to make more hammers, but without the blacksmith.
Compiling data from all relevant papers at the time, Breslow highlighted that any formaldehyde added to a solution containing glycolaldehyde (a two-carbon molecule) was usually turned into more of the latter. He therefore suggested that, at the centre of the formose reaction, there lay a cycle wherein glycolaldehyde duplicates itself by catalysing the reaction between two formaldehyde molecules, with any intermediate compounds exiting the cycle and binding to formaldehyde to form larger sugars. The Breslow cycle, as it is now known, was supposedly the heart of the matter. Breslow was right - to an extent.
Just as the behaviour of a cell can't be explained by a single signalling pathway, the outcome of the formose reaction is too variable to be caused by only one mechanism, particularly if we want to think of it as a template for metabolism. In addition, says Robinson, "There's a lot that has to happen for a chemical system to couple to all the processes in a cell. Just having the cycle is not enough." Most chemists in Breslow's time would have acknowledged this. Nevertheless, subsequent studies have since taken glycolaldehyde as a sort of impetus for molecular diversity, its presence an arbiter for which direction the network would take. Although partially true, the Huck Group saw an alternative part to the system.
According to their results, the Breslow cycle not only generates carbon chains that become longer by formaldehyde addition, but also acts as a source of small molecules that combine together to produce other sugars. Two- and three- carbon molecules (including glycolaldehyde itself) might react to make lyxose or ribulose, with even larger molecules breaking apart to fuel the cycle anew. This would also explain why, when the concentration of formaldehyde is low, diversity increases and more of the largest sugars in the system tend to form. By the same token, the Breslow cycle could be likened to some anabolic cycles in living organisms - pathways that build upon basic building blocks to form complex biological molecules like glucose, such as the Calvin cycle in photosynthesis. Simple speculation, of course, but worth considering in prebiotic chemistry. When I asked her about the subject, Dr Muchowska was partly in agreement.
"The most central metabolic pathways haven't changed for millions of years, and this is what we know from phylogenetic and phylometabolic studies of [ancestral] organisms in the tree of life. If they haven't changed for so long, why would they have changed even beforehand?" However, she was still sceptical about the formose reaction being the entire answer to prebiotic chemistry. At the very least, the study has provided scientists a new route to take in future chemical research, and will inevitably lead to a greater comprehension of the chemistry behind the origin of life.
But that brings up yet another, perhaps more consequential point: why even study prebiotic chemistry in the first place? If we end up travelling between solar systems in the future, I imagine it could play a role in colonising newly found planets. More presently, it might allow cell biology researchers to more easily interpret metabolic pathways in cells, potentially shortening the road to find therapeutic targets. For all that, Dr Robinson emphasised the importance of simply learning about our beginnings, as well as the fascinating opportunity to join chemistry and physics towards a shared goal.
"Two very deep questions we may ask ourselves," Robinson noted in an email, "are 'where did we come from?' and 'are we alone in the Universe?' The disciplines of physics and astronomy have gone quite a ways into answering these questions, but...we still do not fully understand how life could have been created anywhere in the universe. The origin of life, in particular, is a physicochemical phenomenon, meaning that chemistry is uniquely placed to understand how a planet without life can give rise to something living."
References
- Becker, S. et al (2020). Unified prebiotically plausible synthesis of pyrimidine and purine RNA ribonucleotides. Science 366(6461):76-82. Retrieved from https://doi.org/10.1126/science.aax2747
- Surman, A. J. et al (2019). Environmental control programs the emergence of distinct functional ensembles from unconstrained chemical reactions. PNAS USA 116(12):5387-5392. Retrieved from https://doi.org/10.1073/pnas.1813987116
- Lambert, J. B., Gurusamy-Thangavelu, S.A. & Ma, K. (2010). The silicate-mediated formose reaction: bottom-up synthesis of sugar silicates. Science 327(5968):984-6. Retrieved from https://doi.org/10.1126/science.1182669
- Stubbs, R. T., Yadav, M., Krishnamurthy, R., & Springsteen, G. (2020). A plausible metal-free ancestral analogue of the Krebs cycle composed entirely of α-ketoacids. Nature chemistry 12(11):1016–1022. Retrieved from https://doi.org/10.1038/s41557-020-00560-7
- Muchowska, K. et al (2017). Metals promote sequences of the reverse Krebs cycle. Nature Ecology & Evolution 1:1716–1721. Retrieved from https://doi.org/10.1038/s41559-017-0311-7
- Robinson, W.E., Daines, E., van Duppen, P. et al (2022). Environmental conditions drive self-organization of reaction pathways in a prebiotic reaction network. Nature Chemistry 14:623–631. Retrieved from https://doi.org/10.1038/s41557-022-00956-7
- Breslow, R. (1959). On the Mechanism of the Formose Reaction. Tetrahedron Letters 1(21):22–26. Retrieved from https://doi.org/10.1016%2FS0040-4039(01)99487-0